Collaborations and Research Networks
Researchers at LFG are participating in numerous collaborative projects and research networks. The list below summarizes the current third-party funded local and national collaborations in addition to international collaborations of the institute. A summary of completed collaborative and research network projects can be found here.
The institute conducts research projects of all sizes with industry , from small scale materials production and characterisation up to large scale strategic alliances (e.g. in the framework of the Cluster of Excellence EAM). Industrial partners have included BASF SE, Bayer AG, Clariant AG, Evonik Industries AG, Greenerity GmbH, LANXESS Deutschland GmbH and LUM GmbH. Due to commercial sensitivities further information will not be provided here. Many publications of the institute do however contain results of these collaborations. We always welcome new ideas and impulses from industry. Potential industrial partners should address their enquiry to the relevant professor.
Local (third-party funded)
Collaborative Research Center (Sonderforschungsbereich) 1411 (with integrated research training group) - Design of Particulate Products
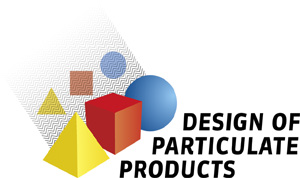
Coordinator: Prof. Dr.-Ing. Wolfgang Peukert
The key objective and long-term vision of Collaborative Research Centre 1411 is the targeted design of particulate products by rigorous optimisation based on predictive structure-property and process-structure functions. Particulate products, as considered in this CRC, consist in the simplest case of dispersed single particles and in more complex cases of hierarchically organised assemblies of particles in the form of supraparticles, thin films or stationary phases for chromatographic separation. We target scientific breakthroughs in the product engineering of nanoparticles with optimised optical properties produced by continuous synthesis directly coupled to property-specific classification of nanoparticles by chromatography. These challenges will be addressed from different perspectives in four strongly interlinked research areas. These will be underpinned by the development of joint methodologies in synthesis, classification, characterisation as well as modelling, simulation, and optimisation.
In CRC 1411 seven research projects and two central projects will be led or co-led by members of the Institute of Particle Technology:
Project A1 – Formation dynamics of coloured particles
Prof. Dr.-Ing. Wolfgang Peukert
The objective is the rigorous design of scalable continuous flow synthesis of silver and gold nanoparticles of various sizes, shapes and composition with the aim to produce particles with desired optical properties. The particle formation dynamics is characterised by multidimensional ensemble methods and by high-end techniques on the single particle level. Predictive one- and two-dimensional population balance models for the seed-mediated growth will be established to tailor the formation dynamics. Finally, the synthesis will be coupled with size- and shape-selective chromatography.
Project A3 – Continuous flow synthesis of patchy particles with designed resonances and interactions
Prof. Robin N. Klupp Taylor (MEng, DPhil (Oxon))
The objective is the deposition of plasmonic patches made of noble metal on dielectric particles. To determine which process parameters lead to a certain degree of coverage and shape of patches, we will perform inline measurements of the kinetics of the redox reactions, the patch nucleation and patch growth during continuous flow synthesis. Thus, we will establish a model for patch formation. Process parameters which lead to excellent optical properties will be identified and the corresponding particles synthesised on the order of few grams and evaluated as pigments. In addition, we will functionalise tailored metal patches on nanoscale core particles in order to endow these with ability to perform anisotropic interactions.
Project A5 – Defined self-assembly of structural colour pigments
Prof. Dr. rer. nat. Nicolas Vogel
The objective is the fabrication of structural colour pigments with optimal colouration. We will use a bottom-up strategy to assemble colloidal particles into defined thin films and supraparticles. We will combine dielectric, absorbing and emitting particles synergistically and control their structure, number ratios and relative positions to produce optimized colouration in close collaboration with mathematical optimization and simulations on structure formation.
Project B1 – Synthesis of hierarchical porous materials for nanoparticle chromatography
Dr.-Ing. Alexandra Inayat, Porous Materials Group, Institute of Chemical Reaction Engineering (external link)
Prof. Dr. rer. nat. Nicolas Vogel
The objective is the development of preparative methods for hierarchical porous materials with controlled pore and surface characteristics, which will be tailored and optimized for application as novel stationary phase materials for the chromatographic separation of nanoparticles. Our preparative approach relies on the synthesis of mesoporous primary particles with defined particle shape and pore size, their controlled assembly into supraparticles with pore size-specific surface properties, and the subsequent conversion of these supraparticles into macroporous zeolite particles with targeted pore diameter, shape and length.
Project B2 – Creation of functional particles and porous structures by spray drying
Prof. Dr.-Ing. Andreas Bück
The objective is the development of a new scalable technology for continuous production of stationary phase materials for use in particle chromatography from suspensions by “spray printing”, i.e. layer-by-layer build-up of functional, porous substrates by individual droplet placement and drying. We will design and implement a new device for layer-by-layer build up. We will perform experiments on solids formation from drying free and sessile droplets and derive dynamic models that will allow describing predictively layer morphology and spatial heterogeneity of the porous substrates.
Project B4 – Fundamentals of nanoparticle chromatography
Prof. Dr.-Ing. Wolfgang Peukert
The objective is the chromatographic classification of nanoparticles regarding size, shape and surface functionality based on an in-depth understanding of the nanoparticle-stationary phase interactions and of the transport processes of nanoparticles in the column and the stationary phase. The interactions will be tailored by index matching and by porosity, size, charge and surface properties of the nanoparticles and the stationary phase material. The retention behaviour of the nanoparticles will be studied and two-dimensional grade efficiencies will be evaluated. Based on novel process concepts, preparative nanoparticle chromatography will be coupled with continuous nanoparticles synthesis.
Project C4 – Multidimensional characterisation of nanoparticle ensembles
The objective is the comprehensive multidimensional characterisation of particle ensembles. We will develop new methodologies for the quantitative characterisation of nanoparticles with respect to size, density, shape and optical properties (extinction and emission) by means of combined centrifugation and gas phase analytics. In addition, we will perform systematic non-ideality studies using analytical (ultra)centrifugation. We will determine the effect of concentration on the sedimentation and diffusive properties of nanoparticles for narrow and gradually more complex size distributions and will further combine these investigations with supplementary, surface sensitive techniques.
Institute members also will contribute to two central projects of CRC 1411:
Prof. Robin N. Klupp Taylor (MEng, DPhil (Oxon))
The iRTG-ParSciTech will provide an organisational framework for doctorates carried out in CRC 1411. It supports the implementation of research projects by means of double supervision, monthly seminars and annual retreats. The qualification concept includes measures which promote technical, organisational and social skills. In particular, interdisciplinarity and internationalisation will be assured through collaborations within the CRC and with guest scientists as well as research stays abroad. Modern methods in particle technology will be taught in dedicated workshops. Finally, the graduate school will, together with other local institutions including the FAU Graduate Center, round off a comprehensive support package for doctoral researchers.
Project Z – Central tasks of the Collaborative Research Centre
The objective is the scientific coordination of the CRC as a whole. Thus, it covers all centralised activities including i) administrative management of all central costs, ii) support of young scientists with special focus on gender equality and reconciliation of family and work life, iii) quality control, iv) internationalisation and v) outreach. The Z project it is the central intersection between all involved institutions, i.e. DFG, the central administration of FAU and with the interdisciplinary centres at the university level.
Cluster of Excellence (Exzellenzcluster) 315 - Engineering of Advanced Materials
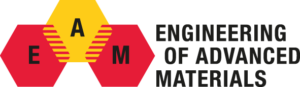
Engineering of Advanced Materials – Hierarchical Structure Formation for Functional Devices
Coordinator: Prof. Dr.-Ing. Wolfgang Peukert
Many LFG researchers have contributed to EAM since its establishment in 2007. Moreover, 2 professorships based at LFG (Prof. Robin N. Klupp Taylor (MEng, DPhil (Oxon)) and Prof. Dr. rer. nat. Nicolas Vogel, the latter succeeding Prof. Dr. Oliver Diwald) were established through EAM funds and are now made permanent at FAU.
Startseite
EAM: A Short Introduction (further details at the Cluster website)
Custom-made high-performance materials are revolutionizing the world of electronics, optics, catalysis and lightweight construction. In Erlangen, materials science innovation starts at the atomic level and influences the entire process chain to achieve macroscopically visible results.
The Cluster of Excellence Engineering of Advanced Materials – Hierarchical Structure Formation for Functional Devices (EAM) is the only interdisciplinary research collaboration of its type in Germany to focus on the investigation of functional materials and their processing at all length scales. Its research centers on the fundamental and applied aspects of designing and creating novel high-performance materials. Situated at the Friedrich-Alexander-Universität Erlangen-Nürnberg (FAU) and involving 9 disciplines covering 3 faculties it is part of the Excellence Initiative of the German federal and state governments.
Particle technology (A1), analytical tools and methods (A2), and multiscale (both temporal and spatial) modeling and simulation (A3) are Research Areas of central importance to all areas of EAM. Their overall goal is to develop common methods for fabricating building blocks (molecules and particles) that are required for formatting structures in the Research Areas B, C, D and E.
These four application-oriented Research Areas are organized in interdisciplinary process chains for the engineering of specific material classes: Nanoelectronic Materials (B), Photonic and Optical Materials (C), Catalytic Materials (D) and Lightweight Materials (E). Demonstrators are developed in these Research Areas in close cooperation with external academic and industrial partners integrated in the EAM.
See also:
Collaborative Research Center (Sonderforschungsbereich) 953 - Synthetic Carbon Allotropes
LFG contributes to project A3 (see below)
Carbon – basis of life, future of materials science
In order to fully exploit attractive applications such as nanoelectronics, sensors, nanocomposites, batteries, and supercapacitors a wide range of fundamental studies and discoveries into the science and technology of synthetic carbon allotropes is still required. It is clear that only an efficient interdisciplinary network of researchers with complementary expertise is the most appropriate tool to achieve important breakthroughs. The scientific aim of the SFB 953 ‘Synthetic Carbon Allotropes’ is to tackle these challenges by making use of the well-recognized expertise in Erlangen in the field of synthetic carbon allotropes through increasing the interactions between the disciplines within a formal environment. The joint effort and the interdisciplinary interaction between chemists, physicists, materials scientists, chemical engineers, and theoreticians forms the basis of our approach to these challenges.
Project A3 – Process Design Strategies for Advanced Carbon Allotropes and Hybrid Nanostructures
Project scientists: Prof. Dr.-Ing. Wolfgang Peukert
We will design advanced and scalable synthesis methods for carbon dots (CDs) and single wall carbon nanotubes (SWCNTs) and will finalize the studies on graphene production by graphite delamination. The solvothermal CD formation mechanisms and dynamics will be studied by in situ spectroscopic methods. The produced CDs will be size-selected and their structure will be carefully characterized, e.g., by a unique combination of Electrospray-Differential Mobility Analysis-Mass Spectrometry and by Multi Wavelength Analytical Ultracentrifugation (MWAUC). SWCNTs will be synthesized in a fluidized bed reactor utilizing tailored non-ferromagnetic catalysts to allow separation of metallic and semiconducting SWCNTs by controlled binding to magnetic nanoparticles after selective functionalization. In addition, we will design synthesis processes for hybrids of carbon allotropes and nanoparticles by wet-chemical methods and surface-assisted growth from the gas phase. For the characterization of the carbon allotropes and their hybrids regarding size and shape, defects as well as functional groups, our already strong tool box will be further expanded, e.g., by new evaluation methods for MWAUC. Interactions of carbon allotropes with nanoparticles will be studied in cooperation with project partners in the SFB 953 for magnetic separation of SWCNTs and in order to tune the electronic, optical and catalytic properties of the hybrid materials for potential applications in solar cells, fuel cells, batteries or in catalysis.
CRC Website
CRC Details (DFG GEPRIS website)
Further information on the CD research project can be found on the LFG research page.
Collaborative Research Center (Sonderforschungsbereich) 814 - Additive Manufacturing
LFG contributes to projects A1, A2, A3 and T1 (see below)
Manufacturing Technologies of the Future
The Collaborative Research Center 814 – Additive Manufacturing deals with the basic research of additive manufacturing processes. In the future, parts should be produced from plastic or metal without specific tooling. Away from rapid prototyping to manufacturing, these technologies are empowered by the CRC 814 to fabricate serial components in the desired quality.
Since 2011, more than 35 scientists are working at the FAU on this large interdisciplinary project. In July 2019, the third funding period started.
Project A1 – Liquid Phase Production of Functional Polymer Particles
Project scientists: Prof. Dr.-Ing. Wolfgang Peukert, Dr. rer. nat. Jochen Schmidt, Dechet, Maximillian (M.Sc.)
The aim of this project is the production of novel polymer particle systems for additive manufacturing processes by applying wet grinding and melt emulsification with integrated surface functionalization. Within the second funding period, the aforementioned processes will be expanded towards filled polymer systems and blends. Furthermore, the energy consumption will be characterized and optimized. Polymer particle systems for the use in the beam melting process (particle size 5 – 20 µm) are obtained by spray drying and spray agglomeration (e.g. composite particles) of the produced product suspensions. These particles with optimized flowability and advantageous packing properties will substantially expand the availability of polymer powder materials for additive manufacturing processes.
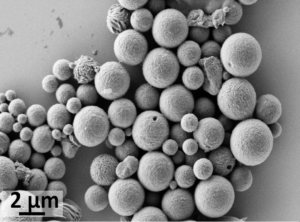
Project A2 – Modification and Functionalization of Powders in Gas Phase
Project scientists: Prof. Dr.-Ing. Wolfgang Peukert, Gomez, Juan (M.Sc.)
The main task of this project is the functionalization of powder materials for additive manufacturing processes using gas phase processes in order to provide tailored process and component properties. The individual sub processes – physical and chemical routes – were successfully investigated within the first funding period. One further aim is to establish processes which are possible to scale-up. Moreover, an integration of single process steps to result in industrial-scale functionalization methods with few junctions is favored. The rounding process of chiseled polymer particles will be expanded by an integrated surface functionalization with nanoparticles and plasma functionalization. The chemical process route utilizes plasma enhanced reactions for a functionalization at moderate temperatures. By an in situ synthesis of nanoparticles, custom-made multi material systems with a high purity can be produced.

Project A3 – Process-Adapted Material Characterization for Selective Laser Beam Melting Processes
Project scientists: Prof. Dr.-Ing. Wolfgang Peukert, Dechet, Maximillian (M.Sc.)
Objective of this project is the comprehensive characterization of powders to be produced and applied within the Collaborative Research Center. The focus is on the convergence of measurement and process requirements. Besides flowability and electrostatic effects, thermal analysis will be further investigated in order to get information about the crystallization behavior of the material. Hereby, very high heating rates as well as low temperature gradients can be represented. A laser-high speed-DSC is established in order to thermally analyze the laser beam melting process in small-scale. Structure property relationships and process-structure-relationships are to be deduced to tailor favorable product properties by optimized process routes.

Project T1 – Quality assurance system for powders used in selective laser beam melting of polymers
Project scientists: Dr. rer. nat. Jochen Schmidt, Hesse, Nicolas (M.Sc.)
Aim of the project is the scientific development of a quality assurance system for polymer powders used in laser beam melting. The quality assurance system is based on two methods. First, an empirical model for ageing of polymers will be established, which considers beside chemical additionally physical ageing mechanism. The model is based on experimentally determined bulk material properties as well as rheological and thermal properties of the present material system. The material model in combination with process data allows the prediction of the ageing state and will lead to a demonstrator software, which will be experimentally validated. Furthermore, a measuring system will be developed, which allows for determination of powder flowability at elevated temperatures and rheological properties of the polymer melt. After validation, the quality assurance system will be transferred to a demonstrator system in close cooperation with the industry partner.
See also:
CRC Website
CRC Details (DFG GEPRIS website)
Graduate School (Graduiertenschule) 80 - School in Advanced Optical Technologies (SAOT)
Prof. Dr.-Ing. Wolfgang Peukert is a principal investigator in the SAOT division on Optical Metrology and has supervised several doctoral researchers on projects related to the nonlinear optical characterisation of particle interfaces. Prof. Robin N. Klupp Taylor (MEng, DPhil (Oxon)) is associate investigator in the SAOT division on Optical Materials and Systems
SAOT: A Graduate School of the German Excellence Initiative
Today, optics is widely regarded as one of the most important key technologies for this century. Many experts even anticipate that the 21st century will be the century of the photon. Optics and optical technologies have impact to nearly all areas of life and cover a wide range of applications in science and industry, e.g. in information and communication technology, in production, process and particle engineering, in medicine, medical technology and life science engineering as well as in energy and environmental technology. Optics is a key underpinning science and baseline technology. Innovations in optics and optical technologies are thus important not only for the subject itself but also for practically all other topics and market segments.
To fully exploit this vast potential fully it is of great importance to adapt the academic education to the requirements of optics and optical technologies. This is a challenging task as optics is extremely interdisciplinary in its application fields. Physicists, chemists, biologists, physicians and engineers working in many disciplines (e.g., materials science, metrology, medicine, manufacturing technology) often use similar optical methods but without close collaboration and thus without knowledge transfer between the disciplines. Because of this diversity and the all-pervasive nature of optics, the efficiency of research and development in optical technology is quite low and must be improved by better academic education. The graduate education of personally and technically highly skilled engineers and scientists in the broad area of optics and optical technologies will achieve further progress in the development and application in this field and especially at the interfaces between the disciplines Physics, Engineering and Medicine. It forms one of the strong pillars for the future scientific and technological innovations and thus for the economic competitiveness of German business and society.
The Friedrich-Alexander-Universität Erlangen-Nürnberg (FAU) provides the infrastructure and the scientific competence in all mentioned disciplines within the Erlangen Graduate School in Advanced Optical Technologies (SAOT) forming a world-leading center for the academic education in optics and optical technologies. SAOT provides an interdisciplinary research and education program of excellence within a broad international network of distinguished experts to promote innovation and leadership in the areas optical metrology, optical material processing, optics in medicine, optics in communication and information technology, optical materials and systems, and computational optics.
See also:
Programme details (DFG GEPRIS website)
Research Training Group (Graduiertenkolleg) 1896 - In situ Microscopy with Electrons, X-rays and Scanning Probes
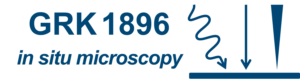
LFG contributes to project B2 (see below)
In situ Microscopy with Electrons, X-rays and Scanning Probes
Research into innovative nanostructured materials is of fundamental importance for Germany’s technological competitiveness and in addressing global challenges, like the development of renewable energy sources. Nanostructured materials are controlled by size and interfaces, which give rise to enhanced mechanical properties and new physical effects leading in turn to new functionalities. The design of novel nanostructured materials and devices such as flexible electronics demands state-of-the-art nanocharacterization tools. In particular, methods based on short-wave radiation (electrons, X-rays/neutrons) or scanning probes are ideally suited to analyze materials at the nanometer and atomic scale. Recently developed in situ capabilities and the use of complementary characterization methods allow unique insights into the structure formation, functionality and deformation behavior of complex nanostructures. These new in situ techniques will be the future key tools for the development of new materials and devices.
Project B2 – Structural changes in nanoparticles under pressure loading
Project scientists: Prof. Dr.-Ing. Wolfgang Peukert, Schwenger, Jan (M.Sc.)
The adhesion and friction of particles is of enormous importance in numerous technological applications. At the same time, the physical processes of contact between particles and substrates, in particular in the lower nm range, are often not completely understood. Contact forces of particles on surfaces and between particles strongly depend on the local contact geometry, in particular the local roughness. These nanostructures in the range <100 nm strongly variable in size. They determine the acting adhesive forces which change as the roughness changes due to elastic and inelastic deformation. The description of these deformations, however, is largely incomplete and is therefore studied in this project.
National (third-party funded)
Priority Programme (Schwerpunkprogramme) 2122 - Materials for Additive Manufacturing
Materials for Additive Manufacturing
This Priority Programme’s main objective is the synthesis of new metal and polymer powders for efficient laser-based 3D additive manufacturing by means of formulations, additivations and (chemical) modifications of both, new and commercial powders. By this, the range of powder materials accessible for laser-based additive manufacturing shall be enhanced significantly. Improving the processability includes requirements like efficient, low cycling time, highly reproducible and precise laser-based additive manufacturing processes. Hence, a recursive research, where the knowledge on materials behaviour during laser-based additive manufacturing is used as input for improved materials design, and vice versa, is preferred. A deepened, preferably predictive, understanding of the materials behaviour during laser-based additive manufacturing by means of suitable analytical and theoretical examination methods is desired.
Participating Project – “Production of SLS particles via liquid-liquid phase separation and precipitation”
Project scientist: Dr. rer. nat. Jochen Schmidt
The overall aim of this project is the development of novel semi-crystalline polymer powders for selective laser sintering (SLS) via a liquid-liquid phase separation (LLPS) and subsequent crystallization for polyethylene terephthalate (PET), polybutylene terephthalate (PBT), polyoxymethylene (POM) and polyvinylidene fluoride (PVDF). Selection criteria for appropriate solvents for LLPS will be deduced by an iterative screening method based on Hildebrand and Hansen solubility parameters. In-situ model LLPS experiments employing dynamic light scattering, optical microscopy and impedance spectroscopy –supported by ex-situ electron microscopy- will allow for deeper insights into the phase separation and particle formation mechanisms. A detailed mechanistic understanding of the underlying mechanism of LLPS, i.e. nucleation, droplet coalescence and growth and elucidation of their dependencies on system composition, time-temperature history will be achieved. LLPS will be implemented on the mini plant scale, where the effect of process parameters (e.g. influence of stirring, cooling regime) on material and bulk solid properties of the obtained polymer particles will be systematically studied. Moreover, in-situ functionalization of the particles with nanoparticulate flowing aids and additive enhancement with thermal stabilizers (antioxidants) will be studied, scale-up criteria will be deduced and powder amounts applicable for studies on SLS processing behavior (several 100 grams to the kilogram scale) will be produced. A detailed structural characterization of the obtained SLS particles with respect to crystallinity, polymorphism, thermal characteristics and morphology will be performed employing amongst others dynamic scanning calorimetry, X-ray diffraction, vibrational spectroscopy (IR, Raman) and electron microscopy. The effect of process parameters on molar mass distributions of the polymer and melt viscosity will be assessed by gel permeation chromatography and melt rheology. Bulk solid characteristics such as the product particle size distribution and the powder flowability which are seen to be most important for processing will be characterized by laser diffraction particle sizing, respectively shear testers and powder application model experiments. The gained understanding of the structure-property relationships and the information on SLS processability provided by the cooperation partners will be utilized for further LLPS process optimization to tailor the desired properties of the novel SLS powders. Novel SLS powders with improved material behavior, i.e. chemical resistance (PET, PBT, PVDF), impact resistance (PBT), high stiffness, excellent dimensional stability (PET, PBT, POM) shall be developed widening the field of application of SLS-manufactured parts.
See also:
Priority Programme Website
Priority Programme Details (DFG GEPRIS website)
Priority Programme (Schwerpunktprogramme) 2045 - Highly specific and multidimensional fractionation of fine particle systemes with technical relevance (MehrDimPart)
The aim of the priority programme is to develop a new class of fractionation methods for highly specific and multi-dimensional particle properties in the size range <10 μm up to a TRL 4-5, by inducing separation features in the particle systems from the outside, implementing the processes with extreme operating parameters, the throughput of analytical methods is increased by orders of magnitude and new physical, chemical, thermodynamic separation principles are introduced.
Participating Project – “Continuous size- and shape-selective separation of nanoparticles”
Project scientist: Prof. Dr.-Ing. Wolfgang Peukert
The objective of this project is the establishment of technical relevant scalable multidimensional separation processes for particles < 100 nm in at least one dimension. This requires the characterization of particle size and shape in suspension as well as the development of optical inline-detection processes which allow the transformation of measured UV/Vis extinction spectra into particle size distributions. The multi-wavelength analytical ultracentrifugation (AUC) will be used as a reference method because among the known particle size measurement methods it is the most reliable method to analyze nanoparticles regarding size and shape anisotropy down to sizes < 1 nm. The development of technical separations for spherical and anisotropic nanoparticles is highly challenging. First, we will focus on the development of a chromatographic size-selective fractionation process for spherical noble metal (Au) and semiconductor (ZnO) nanoparticles. The interactions between the particles and the stationary phase will be investigated in detail. In the next step we will study methods for shape-selective separations. In particular, methods for the fractionation of 2D-materials such as MoS2 with respect to diameter- and thickness will be studied. The potential of antisolvent precipitation, electrophoresis and of chromatography for continuous classification will be investigated in detail.
See also:
Priority Programme Website
Priority Programme Details (DFG GEPRIS website)
Priority Programme (Schwerpunktprogramme) 1934 - Dispersity-, structural- and phase-changes of proteins and biological agglomerates in biotechnological processes (DiSPBiotech)
This Priority Programme’s main objective is the development and use of innovative analytical and numerical methods for the investigation and description of biotechnological processes at the protein, bioagglomerate and process environment level. This will allow a much better knowledge and prediction of the effect of mechanical, chemical and thermal interactions on the denaturation of the proteins along the process chain as well as the formation and structure of the bioagglomerates and thus the yield and quality of the products.
Participating Project – “In situ Studies of Structure Changes of Biomolecules at Interfaces and Under Fluidmechanical Stress”
Project scientist: Prof. Dr.-Ing. Wolfgang Peukert, Uttinger, Maximilian (M.Sc.), Private: Guckeisen, Tobias
In this project, changes in both molecular structure and dispersive properties of biomolecules are studied under relevant stress conditions and structure-process correlations are extracted. We expect that for each biomolecule unique critical stress conditions e.g. maximum shear and tensile stresses can be found which either lead to structure changes, to changes in the dispersive properties or both. From the determined maximum stressing parameters, optimal conditions for process engineering of biomolecules are to be deduced, where structure changes etc. can be avoided. Since in many applications a complex interplay between fluid mechanical and chemical (pH, ionic strength, etc.) stressing conditions with and without the presence of fluid/gas and fluid/solid interfaces exists, it is necessary to perform experiments where the latter can be isolated from each other. For that reason we will follow an hierarchical approach where the situation e.g. during filtration , within stirring tanks etc. is transferred to model experiments. For that reason the project is divided into the following working packages (WP):WP1. Influence of well-defined fluid mechanical stress conditions on changes in molecular structure and dispersive nature of biomolecules as a function of pH, ionic strength and species as well as a function of biomolecule concentration.WP2. Fluid mechanical stress in micro channels with different geometry studied with high resolution in situ microscopy and spectroscopyWP3. Interface induced changes and identification of material properties and chemical environment with in situ nonlinear optical spectroscopy.
See also:
Further project details in LFG subgroup “Surfaces and Interfaces”
Priority Programme Website
Priority Programme Details (DFG GEPRIS website)
Priority Programme (Schwerpunktprogramme) 1679 - Dynamic Simulation of Interconnected Solids Processes
The material and the energy conversion of technical processes often consist of many individual substeps, which are interconnected by material, energy and information flows. The networking of each component has significant influence on the dynamic behavior and the stability of such processes. For the design and optimization, especially with respect to the reduction of energy and raw material demands, not only the individual components should be simulated, but also the dynamic behavior of the total process. This is state of the art in fluid process engineering as there are several tools commercially available for dynamic flowsheet simulation. However, such tools and dynamic models, that allow for a dynamical flowsheet simulation independent of the considered system, are missing for the field of solids process engineering. The reason for this is the complex description of solids with their multivariate disperse parameters and the associated processes for the conversion of solids. The main focus of the Priority Programme is the development of numerical tools of the dynamic simulation of interconnected solids process. Therefore, the dynamic models of each machine and apparatuses of solids process engineering should be formulated and implemented.
Participating Project – “Flowsheet simulation of integrated precipitation processes examined for the case of a modular micro reaction setup”
Project scientists: Prof. Dr.-Ing. Wolfgang Peukert, Prof. Dr.-Ing. Doris Segets
A wide range of particulate materials is produced in liquid phase. Due to its modularity which enables high flexibility and continuous operation, micro reaction technology (MRT) is playing an increasingly important role. Superimposing mechanisms make precipitation highly dynamic and sensitive against even slight variations in process parameters. The target of the present project is the development of an extensive and generally applicable module describing precipitation processes in MRT setups for the dynamic flow sheet simulation environment Dyssol. Besides simulation, process optimization is an important aspect in today’s engineering and is therefore included in this project. Several different inorganic material systems are used throughout this project for model development to ensure flexibility with respect to apparatus and reaction. Models describing sub-processes such as mixing and complex formation as well as several competitive solid formation processes are closely linked. The commonly used particle size distribution (PSD) as description of the disperse phase is often insufficient to account for all relevant ensemble properties. Therefore, the tool is extended to allow for two disperse properties (2D) being followed in parallel, such as core size and shell thickness of core-shell particles or shape anisotropy based on anisotropic growth and oriented agglomeration. The formation of multiple solids is treated via parallel balancing over multiple phases by a reduced, numerically efficient population balance model. According to the categorization into slow, reaction controlled and fast mixing controlled systems that has been applied throughout the whole project, an existing flow sheet module describing Ostwald ripening in MRT setups is combined with the work on fast precipitation systems. Based on the time scale of the dominating processes, the most appropriate method and the corresponding solver is selected to guarantee high numerical stability and short computational time. Finally, a module designated for process optimization of MRT setups is developed. While models for standard flow sheet simulation only consider the impact of the total of all process parameters on the disperse properties, optimization requires the impact of each process parameter in terms of the respective adjunct. The possibility of applying a gradient-based method with its faster convergence compared to gradient-free methods allows the optimization within a flow sheet simulation framework. Hence, after finishing this research project, dynamic precipitation processes in continuous MRT setups are possible to be modeled and optimized in flow sheet simulations.
Participating Project – “Impact comminution in jet mills”
Project scientists: Prof. Dr.-Ing. Wolfgang Peukert, Prof. Dr.-Ing. habil. Karl-Ernst Wirth
The objective of this project is the experimental analysis of two-phase flow in fluidized bed jet mills and the development of a related flow-sheet model for implementation in Dyssol. The fluidized be opposed jet mill is particularly interesting because three key unit operations, namely impact comminution, pneumatic transport and classification with the integrated impeller wheel classifier, are combined into one apparatus. In the first funding period, the stressing conditions experienced by the particles in the multi-phase jets were characterized in detail. For this purpose, different experimental set-ups were used to investigate the fluid mechanics in the multiphase jets. The particle velocity in both the subsonic and choked flow regimes was measured in a semicircle plant using Particle Image Velocimetry. In this way, the kinetic energy required for particle breakage was calculated. The solids volume concentration in the jet was measured via capacitance probes. Moreover, flow pattern could be analyzed noninvasive by x-ray computer tomography.Additional grinding kinetics have been determined in a commercial fluidized bed opposed jet mill. Based on this, a simple model for the grinding kinetics was established, which combines fluid mechanics and material specific breakage probabilities. Furthermore, a shortcut model for the comminution unit within the mill was developed. This model will be extended by further experiments for different process and geometric parameters. Main focus is on the two most important parameters: the particle size distribution and the holdup, which continuously change during dynamic processes. The experimental characterization of stress number and stress energy distributions has been realized in a stirred media mill for two-sided stressing by a novel method based on mechanically well-defined spherical metal particles, and will be transferred to one-sided stressing in the fluidized bed mill. Mechanically fully characterized metal particles will be stressed in the mill. Subsequent image analysis of the stressed and deformed particles allows the determination of the number of stress events and the absorbed energy. Finite element simulations in combination with SEM supported compression experiments can relate the shape of the compressed particles to the volume-specific energy absorption. Material functions for one-sided impact stressing will be measured by the newly built single particle impact apparatus. The breakage probability and breakage function of limestone, glass and alumina particles (< 40 µm) will be systematically measured.
See also:
Priority Programme Website
Priority Programme Details (DFG GEPRIS website)
International (listed according to LFG Professor)
Prof. Dr.-Ing. Wolfgang Peukert
Borris Demeler, University of Lethbridge, Canada
- Analytical ultracentrifugation
Juan Fernandez de la Mora, Yale School of Engineering & Applied Science, USA
- Electrospray technology
Yongshen Han, Chinese Academy of Science, Institute of Process Technology, Beijing
- Flow synthesis of silver particles
Hidehiro Kamiya, Tokyo University of Agriculture and Technology, Institute of Global Innovation Research, Tokyo
- Surface functionalization and product design
Luis M. Liz-Marzán, Center for Cooperative Research in Biomaterials, San Sebastian, Spain
- Analysis of plasmonic nanoparticles
David Pui, University of Minnesota, USA
- Filtration of nanoparticles
Walter Stafford, Harvard Medical School, Boston, USA
- Models for analytical ultracentrifugation
Chika Takai-Yamashita, Gifu University, Japan
- Synthesis of hollow particles
Yasushige Mori, Doshisha University, Kyoto, Japan
- Classification of nanoparticles
Prof. Robin Klupp Taylor
Chika Takai-Yamashita, Gifu University, Japan
- Metallization of nanostructured silica particles
Satoshi Watanabe, Kyoto University, Japan
- Continuous flow microreactors for metal coated particles synthesis
- In situ linear and nonlinear optical measurements of growing core-shell particles
Prof. Nicolas Vogel
Joanna Aizenberg, Harvard University, USA
- Liquid-infused repellent surfaces
Nick Boechler, University of California, San Diego, USA
- Phononic properties of self-assembled materials
Gilles Bourret, Salzburg University, Austria
- Colloidal lithography and the fabrication of silicon nanowire arrays
Martin Buzza, Hull University, UK
- Theory of colloidal self-assembly
Syuji Fujii, Osaka Institute of Technology, Japan
- Particle systems for the design of liquid marbles
Mathias Kolle, MIT, USA
- Structural color in self-assembled materials
- Light guidance in polymer fibers
Eric Mazur, Harvard University, USA
- Plasmonic surfaces for optoporation
Satoshi Watanabe, Kyoto University, Japan
- Fabrication of metal-organic framework supraparticles